To begin with, I remind you that I am a geologist, not a nuclear physicist, but I do have some basis for understanding and divulgation as well. I want to do this because as a person of science, I should not have also believed certain scientific inaccuracies that have become almost stereotypes. One only has to delve a little deeper into the subject (from scientific sources) to discover that it is not all as dark as it is painted. We are usually afraid of things we do not know. Culture is freedom.
Let’s start with clarifying: in nature we observe 4 types of energy, electromagnetic energy, gravitational energy, weak nuclear energy and strong nuclear energy. The first two we can experience in everyday life. Light itself is an expression of electromagnetic energy, but so are wifi connections and radio waves. Nuclear energies are less visible because they act within atoms.
Matter is made of atoms of different elements combined to form compounds. An atom is made up of subatomic particles such as protons and neutrons, which are amassed in the nucleus, and electrons, which orbit the nucleus at great distance and speed. Electrons have tracurable mass compared to protons and neutrons. They have a negative electric charge, while protons have a positive charge. Neutrons are so called because they have no charge (they actually consist of a proton and an electron, so the charge is canceled out).
Each element has its own typical characteristics due to the number of protons in its nucleus: tell me how many protons you have and I will tell you who you are. Hydrogen has only one proton, helium has 2, oxygen 8, carbon 6, gold 79, uranium 92. But think that protons, since they all have a positive charge, should repel each other. How do they all stick together in an atomic nucleus? In fact, normally they would repel each other, but at very small distances (one millionth of a millionth of a millimeter = 10-12 mm) the forces of attraction that hold the atomic nucleus together come into play. Bringing protons so close together is something that can happen only in the core of stars or by the enormous energies created when they explode as supernovae ( it is called nuclear fusion). This is how all atoms were formed, even those of which we ourselves are composed.

Schematic representation of a helium atom, with 2 protons, 2 neutrons and 2 electrons.
Neutrons are about the same number of protons but there are some atoms of the same element that have more or fewer – they are called isotopes. Let’s take an example: we said carbon has 6 protons in the nucleus. Usually we would expect it to also have 6 neutrons, for a total of 12, which we call the atomic weight of carbon (specifically “carbon 12” = 12C). But in nature not all carbon atoms are made equal, some (1 in a trillion) go so far as to have 8 neutrons for a total of 14 particles in the nucleus; carbon 14 (14C) is radioactive: one of the neutrons loses the electron it contains turning into a proton. So the atomic number goes from 6 to 7, transforming the atom into nitrogen (14N). This transformation releases energy in the form of high-frequency electromagnetic waves (like light but at frequencies so much higher that we do not see them and, like X-rays, they pass through us). We are made of carbon and a percentage of it is 14C.
The neutrons are there in the nucleus because by adding electrically neutral mass they help keep it stable, that is, keep protons from escaping. But they don’t always succeed, and that is exactly what happens to the largest nuclei, those with more than 83 protons: they lose protons thus turning into a different element. Uranium, for 92 protons in the nucleus has 146 neutrons (92+146=238U). Uranium 238 is the most widespread in nature and is unstable, decaying slowly by losing two protons and two neutrons, thus transforming into an atom that has 90 protons in the nucleus, thorium (234Th). Just think that a mass of uranium 238 takes more than 4 billion years to turn half into thorium. Therefore, its radioactivity (the energy it naturally emits by transforming) is quite low; it is part of the Earth’s natural radioactivity background with which life developed and evolved. It is absolutely harmless. In fact, it warms us: more than 40 percent of Earth’s internal heat (on average, the temperature rises 30°C per km of depth) is due to the decay of uranium – our planet keeps itself warm with nuclear energy!
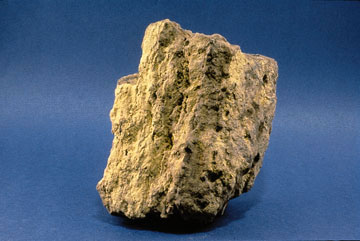
Raw uranium
0.7 percent of all the uranium in nature is its 235U isotope, which with three fewer neutrons is a little more radioactive than uranium 238. But not so much as to cause us problems. It is another of its characteristics that instead allows us to use it to generate energy. It is the only “fissile” element in nature, meaning that if we bombard its nucleus with neutrons, it tends to break into several parts. The nucleus of uranium 238 doesn’t want to know about it; on the contrary, the neutron is welcome in its nucleus, it absorbs it and becomes 239U! When an atomic nucleus splits (nuclear fission) it emits energy, and that is the nuclear energy we talk so much about.
In summary: naturally occurring uranium is almost all 238U. It will decay radioactively very slowly (in over 4 billion years, a bar of uranium will have become half thorium). So if we take a bar of uranium and use it as a paperweight on our desk, nothing much happens to us. It’s a different matter if we breathe in uranium dust in a mine; it’s as toxic as other non-radioactive metals, but uranium gets fixed in the bones and stays there decaying, emitting radiation — not pretty. But as it stands, inert on the desk, it does no damage. It will emit small particles of its decay products, including radon for example, an odorless, heavier-than-air gas that is far more dangerous in cellars and basements of houses in volcanic areas instead, but I don’t see people tearing their hair out over it. Let’s just say that it is still inadvisable to keep it in the house as a paperweight because it can always fall, break, creating chips and dust that is best not to be inhaled or ingested – it was to give the idea…
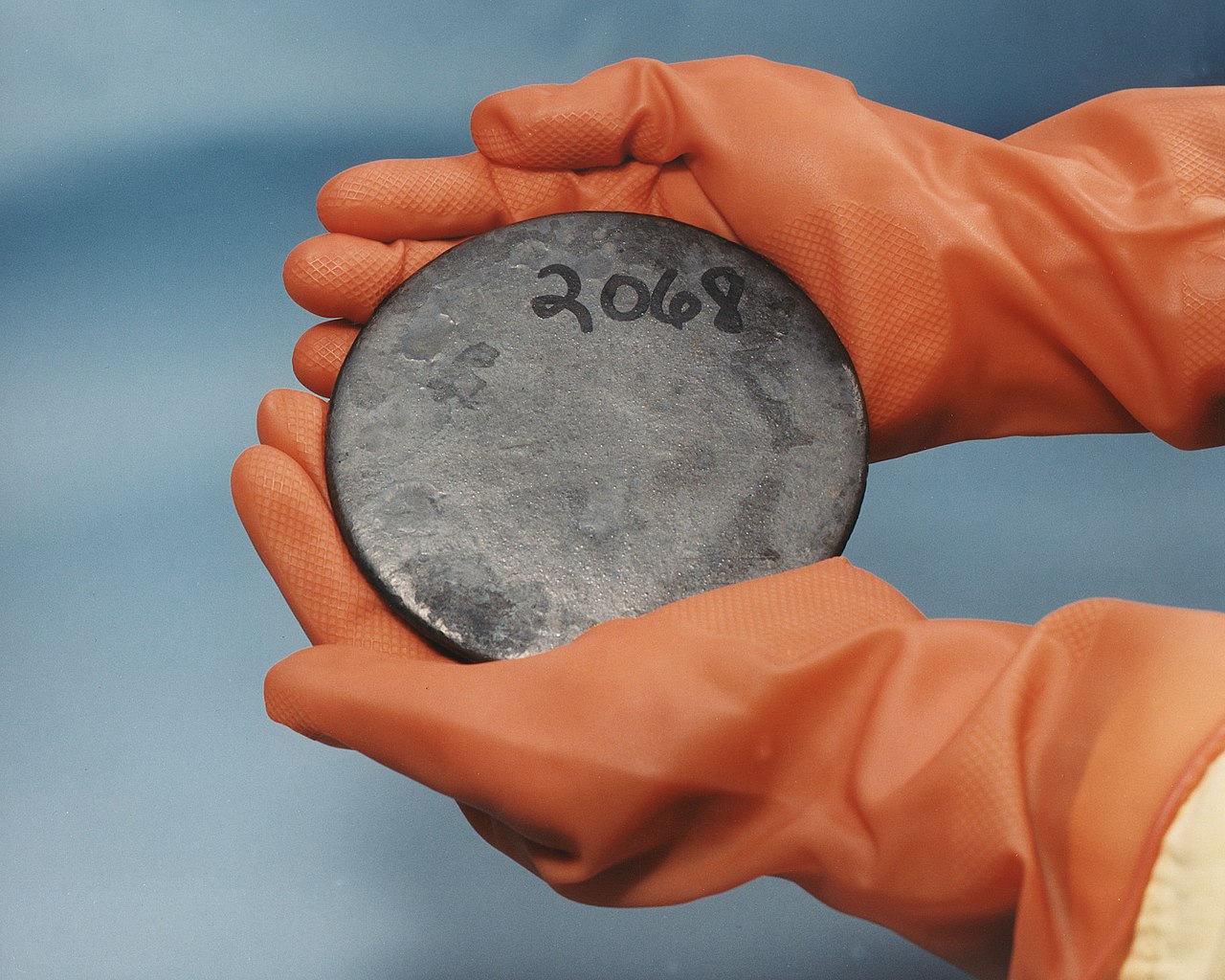
Highly-enriched uranium
However, 0.7 percent of that bar will consist of 235U. If we want to use this bar in a nuclear power plant to create energy, we have to trigger a process called a chain reaction: we bombard the bar with neutrons in the hope of catching as many 235U nuclei as possible. Of course, with the 0.7 percent that there is on average in it, it won’t be easy. In fact, nothing would happen (you practically miss them!). You have to enrich the uranium with 235U, more than is normally found in nature. Once we “add” more 235U to our rod (up to about 3-5%, taking it away from another mass of uranium that will then become “depleted,” i.e., almost pure 238U, the chance of “hitting” its nuclei increases. For every 235U nucleus that is shattered there will also be quite a number of neutrons running around and each of them will have a good chance of bumping into another 235U nucleus and so on – if the percentage of 235U is right, a chain reaction is started that will not stop until 235U is depleted (i.e. until it drops below the percentage that would mantain the chain reaction). At this point our rod will be radioactive as long as the reaction lasts; but also afterwards because many of the elements (the radioactive waste) produced by breaking down the 235U atoms will continue to decay and thus be radioactive, although only for a few years or decades. There is only a problematic one, plutonium (a radioactive element that exists precisely because it is generated in this way), for which it takes centuries and must therefore be disposed of by properly isolating it or reusing it. Today we have the technology to recycle plutonium. It is also fissile so it can trigger chain reactions: we can put it back into the cycle avoiding the problem of disposal. Plutonium would then be broken down into elements with shorter decay, therefore better manageable (it’s self-sustaining fission, with one of its own waste products).

Nuclear power plant (the smoke coming out of the funnels is water vapor, not radioactive gas đ )
Unenriched uranium was once used in power plants. The problem is that it likes neutrons, and if the neutrons are not energetic enough, that is, fast, it absorbs them without getting broken up. But if the neutrons are too fast there is a risk that they will not impact the nuclei passing through the uranium mass unharmed. This means that fewer neutrons would remain available in the hope that they would hit the few fissile 235U nuclei of unenriched uranium. They used to slow neutrons down to the ideal speed by passing them through a material with little neutron-absorbing capacity so that they would not lose too many in an attempt to moderate their speed. The “moderator,” precisely, was heavy water. Water is composed of hydrogen and oxygen (H2O). Hydrogen has only one proton and normally only one neutron. One of its isotopes has 2 neutrons and is called deuterium. Heavy water is made from this type of hydrogen (D2O) and therefore, since it already has one excess neutron, it is unlikely to absorb any more. Thus heavy water lowered the total neutron absorption of the system allowing the use of unenriched uranium. But it is difficult and expensive to isolate heavy water from normal water, so today we prefer to enrich uranium.
Depleted uranium, on the other hand, is a very strong metal and is used for armaments, missiles, bullets, and tank coatings. Since it is high in 238U (because it is depleted of 235U) it is even somewhat less radioactive. So what is left over from the enrichment process can be used that way. Clearly, if you are hit by a bullet or shrapnel of depleted uranium, some of the uranium particles can remain in circulation in the body and create health hazards as they decay, albeit slowly.

Depleted uranium bullet
One of the ideas we normally have is that these are problems of nations that have nuclear power plants. No. It’s a problem of any nation where nuclear medicine and nuclear physics research is done. These activities, which are fundamental even if you just think about health, the diagnoses they allow, generate radioactive nuclear waste that has to be disposed of. Countries have been disposing of them for decades, ever since in the hospital we can do scintigraphy (they inject us with a radioactive material that once in the bloodstream can be seen very well in the X-rays). The decay products continue to decay in turn and must be recycled (reused as long as they continue to emit) or disposed of (isolated in special containers). These are neither impossible quantities nor particularly complex technologies. And it has been done routinely for decades without any harm to health or the environment.
Another misleading idea we get if we do not know the subject well is to equate a nuclear power plant to an atomic bomb. It is difficult enough to trigger a chain reaction in uranium to generate sufficient energy to spin a power plant turbine. To have a chain reaction that generates enough energy to explode as a bomb requires enriching uranium of its 235 isotope well above the percentage for nuclear power plants! You need over 90% of 235U versus 3-5% needed for a power plant. Far more work and expenses! In other words, a bar of enriched uranium for use in a nuclear power plant could never make it to explode; it does not have enough 235U. Building nuclear power plants and building atomic bombs are two different technologies that have in common the use of uranium 235; the enrichment process is the same, and those who have this technology can also use it to enrich uranium to a “weapon-grade” level. But a nuclear power plant simply can’t go off in a nuclear explosion.